Sulfur Energy Storage Could Provide Low-Cost Green Propulsion
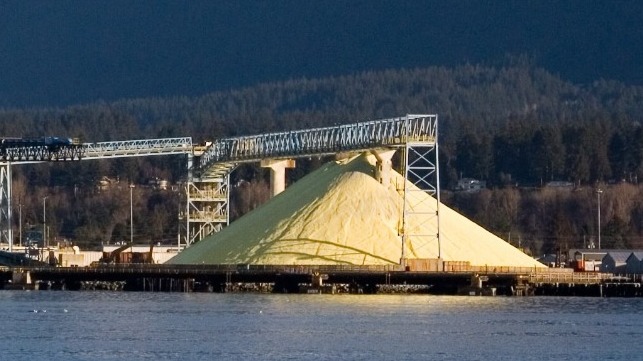
Advances in the chemistry of thermal reactions involving sulphur, oxygen and hydrogen can generate temperatures of 900 to 1200-deg C that can sustain the operation of externally heated gas/air turbine engines. The byproducts of the chemical reaction can be decomposed using intense heat and reused for multiple repeat cycles.
Introduction
The history of thermal energy storage for mobile applications dates back to the 1800s, involving a submarine that carried an insulated tank of saturated water at over 100-pounds/square-inch at 327 deg F. Modern solar thermal power plants use molten salt thermal storage at 1020 deg F. While latent-heat-of-fusion metallic compounds and mixtures combine very long usable service life with high storage density, thermal energy storage duration is short-term. While the reaction of mixing certain chemicals produces heat, it is possible to store the chemicals separately over extended duration until heat is needed.
Heat-producing chemical reactions involving some chemicals are actually reversible. A consortium based in Germany known as Pegasus is undertaking pioneering research into using sulphur thermochemistry to produce heat capable of sustaining the operation of externally-heated turbine engines. The reaction is intended to be reversible, with an external source of heat (like a shoreside nuclear reactor) decomposing the reaction byproducts into a form that can repeatedly be reused.
Heat of Formation
The combustion of hydrocarbon liquid fuel with oxygen releases heat and produces new compounds such as steam and carbon dioxide. While electrolysis can separate hydrogen from oxygen, only photosynthesis from the natural world can separate carbon from oxygen. There are heat-producing chemical reactions that produce new compounds that can subsequently be thermally decomposed back to the original constituents and made available for future reuse to generate heat. Such is the case for mixing compounds of hydrogen, sulphur and oxygen, with sulphuric acid (H2SO4) first being decomposed into water (H2O), sulphur dioxide (SO2) and oxygen (O2).
A second stage involves sulphur dioxide being decomposed to produce elemental sulphur, which is then stored.
When energy is desired, the reaction of sulphur with oxygen produces temperatures of up to 2,200 degrees F, with the heat of reaction being sufficient to sustain the operation of an externally-heated turbine engine connected to a bottom-cycle steam engine. If successful and viable, the research efforts of the Pegasus consortium have potential to form the basis of future ship propulsion.
Ship Propulsion
Research from Pegasus suggests that 50 hours of operation of an engine of 67,000 horsepower would require 5,000 metric tonnes of sulphur and 16,000 metric tons of sulphuric acid. A ship weighing 200,000 tonnes requires 100,000 horsepower to sail at 25 knots. Slowing the ship to 12.5 knots would reduce power requirement to 10,300kW or 13,800 horsepower, allowing for up to 235 hours of engine operation and a sailing distance of over 2,500 nautical miles. Following arrival at port, the spent compound would be transferred to barges that would sail to nearby a high-temperature nuclear power plant, where the compound would be thermally decomposed for reuse.
While still at port, other barges would arrive to deliver sulphur and sulphuric acid from a thermal recycling facility, to provide power for the next voyage. Future ships powered by sulphur thermal technology would sail between ports located near to a high-temperature nuclear power plant, or a solar thermal power plant capable of operating at the temperatures required to thermally decompose sulphur dioxide and recycle sulphur compounds for future application. While the original research focused on stationary thermal energy storage application, the sulphur thermal storage technology has potential in a mega-size transportation vehicle such as a ship.
Cost Issues
While gasoline might contain as much as 45,000 kilojoules per kilogram, sulphur holds some 12,500 kilojoules per kilogram. During early 2024, gasoline incurred a cost of $0.11 per kilowatt-hour while sulphur incurred a cost around $0.02 per kilowatt-hour. While it is presently not possible to recycle the combustion byproducts of gasoline to produce new gasoline, it is technically possible to thermally decompose sulphur dioxide to produce sulphur for future reuse generating heat. In ship propulsion, sulphur technology would be restricted to ships that sail at low speed and might make frequent stops to refuel.
There would be potential for ships powered by sulphur technology to sail coastal services and be assigned to short-sea shipping operation in regions of the world where high-temperature thermal technology would be available. Sulphur-based thermal storage technology would likely have to prove itself in stationary application, involving solar thermal power plants and high-temperature nuclear power plants where sulphur technology might be recycled during overnight off-peak periods. Once proven in stationary application over an extended duration including involving seasonal energy storage over extended time duration, experiments involving ship propulsion might begin.
Conclusions
While the maritime sector is subject to governmental pressure to reduce carbon exhaust emissions, some carbon reduction technologies incur high cost and raise the operating cost of a ship. Other evolving technologies actually reduce ship operating cost. At the present state of research, sulphur thermal storage technology offers the possibility of simultaneously reducing carbon emissions and overall operating cost. The ideal scenario would involve a single thermal power plant providing for the operation of a small fleet of vessels powered by sulphur thermal storage technology while engaged in coastal shipping and short-sea shipping.